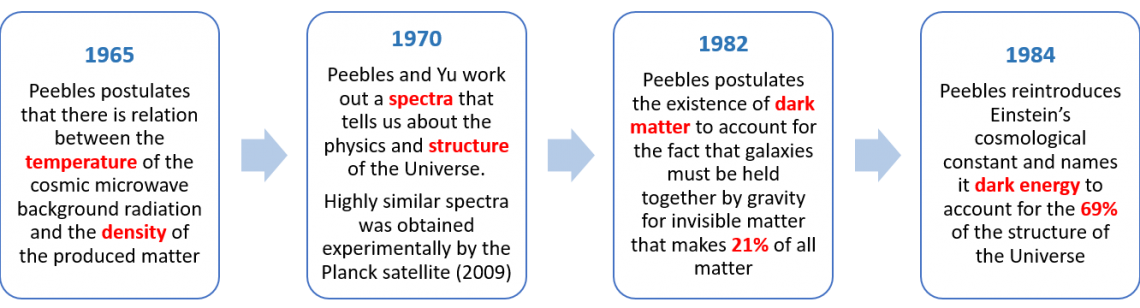
Nobel Prize in Physics, 2019 Part I: James Peebles
Have you ever wondered who was the first person to think that the Universe might be expanding? No, it is not Einstein. The first man to think of an evolving – not static – Universe, was the American writer Edgar Allan Poe in his prose poem Eureka (1848), where he suggested that everything might have started from a “primordial particle”, which then exploded and expanded.
Nevertheless, when it comes to Physics, we Physicists value original ideas immensely, but eventually can disregard any seemingly brilliant idea if it is not supported via proper theoretical framework, mathematical description, analytical models, and experimental confirmations. When it comes to the idea of an expanding Universe, all of these came almost a century after Poe’s revolutionary idea. The foundations of physical cosmology were set in the 1960s and by the mid of the 1980s, these foundations gloriously transformed into a palace of knowledge for our Universe. Large portion of the relevant theories were developed by James Peebles who, with his creative and bold questioning of the Universe’s structure and history, set the cosmological field on the Physics map and paved the way for a new world of knowledge, freed from the chains of legends and myths. This year, for his life-long dedication and “contribution to our understanding of the evolution of the universe”, James Peebles is awarded one half of the Nobel Prize in Physics for his “theoretical discoveries in physical cosmology”. In this post, I will try to refer a bit to the history of cosmology and explain why and how he came to be undoubtfull deserving of the highest award in the scientific world, while in the second part of this post I will talk about Michel Mayor and Didier Queloz – who were both awarded with one quarter of the Nobel Prize in Physics “for the discovery of an exoplanet orbiting a solar-type star”.
Physical cosmology and James Peebles
“The eternal mystery of the world is its comprehensibility” – said Alert Einstein in 1936, 20 years later after he formulated his General Theory Of Relativity and set the foundations of the modern cosmology. The idea of the Big Bang – a time when the Universe was extremely dense and hot – was born. After some 400 000 years, the Universe cooled down and electrons were finally able to combine into nuclei and form the first atoms of hydrogen and helium. Photons started to move freely because there were no charged particles left to interact with them, and consecutively, light started to travel through space, or as many would like to say: “the Universe became transparent to light”.
But, how can we actually know that the Big Bang happened and how can we find the fingerprints of these first moments of the Universe? This became possible in 1965, when Arnold Penzias and Robert Wilson described in a paper the existence and the identification of the cosmic background radiation (or, cosmic microwave background CMB) – a groundbreaking discovery for which they were awarded with a Nobel Prize in 1978. This radiation is a remnant electromagnetic radiation from the early stage of our Universe and it is therefore, the oldest electromagnetic radiation to our knowledge. It cannot be observed with a traditional optical telescope, but if a sufficiently strong radio telescope is used, then a faint signal – almost like a background noise – is observed. It was exactly this “noise” that Penzias and Wilson observed in 1965 with their antennas. Soon after these measurements, Penzias and Wilson familiarized themselves with the work of Peebles who, together with Dicke, Roll, and Wilkinson, stated in the paper “Cosmic black-body radiation” the following crucial point: there is a connection between the radiation’s temperature and the density of the matter, which in return determines how much helium is produced. Peebles formulated the idea that the radiation’s temperature could provide insight into how much matter was produced into the Big Bang, and also understood that this can later explain how matter could cluster up to form our Universe as we know it. Later in 1965, Peebles set the starting point of physical cosmology in his paper “The blackbody radiation content of the Universe and the formation of galaxies” where he postulated that “a critical factor in the formation of galaxies may be the presence of a blackbody radiation content of the universe” and that without it, the Universe would have cooled down to a uniform dullness, instead to the wonderful and mysterious vastness we see today.
By 1967, Rainer Sachs and Arthur Wolfe predicted anisotropies in the background radiation – small temperature fluctuations in the blackbody radiation left over from the Big Bang. In 1970, Peebles and Yu published a paper in which by using numerical methods, they presented a power spectra of density fluctuations and stated that the actual size of the scales on the spectra is affected by the geometry of the Universe. This spectra is even more significant nowadays when we can see how similar it is to the experimentally-observed spectra by the Planck satellite launched ten years ago, for which I will talk a bit later in this article. However, by the 1980s, the cosmological calculations – which were based on the idea of an open universe – were not predicting anisotropies compatible with the observations. The problem was: if the Universe was indeed open, then the anisotropies should have been detected by then. Furthermore, problems with the galaxies formation and the amount of ordinary matter also appeared when the measured rotational speeds of galaxies indicated that the galaxies must be held together by gravity of an invisible matter.
In order to account for this gravitational force, Physicists postulated the idea that neutrinos or other weakly-interacting particles represent a hot dark matter that helps the formation of Universe as we know it. Sadly, the neutrinos’ low mass that enables them to move almost with the speed of light prevents them from gluing matter together. Then, in a paper from 1982, Peebles reached his second breakthrough by postulating the idea of a cold dark matter that behaves fundamentally different that ordinary matter – namely, that it interacts with gravity, but it does not behave the way atoms do. Through the introduction of this non-relativistic, cold dark matter, Peebles succeeded to couple anisotropies in the CMB to our observable structures in the Universe and his predictions on the temperature anisotropies were in agreements with the measurements by the Cosmic Background Explorer (COBE) in 1992.
However, dark matter could not have been the end of the story, since it was found that ordinary matter made up only 5% of our Universe, dark matter 26% and thus, there was 69% missing “matter”. Peebles – once again – came up with a revolutionary idea and reintroduced Einstein’s cosmological constant. Einstein introduced this constant when he developed his theory of General Relativity, but when the theory yielded the conclusion that the Universe was expanding, Einstein added a term – the cosmological constant – in his equations to counterbalance the effects of gravity and make the Universe stand still. However, when in 1929 – almost a decade after the Theory of General Relativity was postulated – Edwin Hubble and his observations lead to the general conclusion that the Universe was indeed expanding, Einstein named the cosmological constant “the biggest blunder in his life” and sent it into oblivion for more than five decades. In a 1984 paper, Peebles argued that it is reasonable to consider the gravitational constant when we think of the structure formation in our Universe. He liked the idea of a flat Universe with a critical density – suggested by the novel theories of inflation – but the problem was that the measured mattered density was too small for the Universe to be flat. Thus, Peebles predicted that the cosmological constant, in combination with the cold dark matter, would make up for the missing matter density and solve the so-called age problem, where the estimated age of the oldest stars exceeded the age of the Universe. The cosmological constant is, more precisely, the vacuum energy, and thus, it represents a constant energy density which is unaffected by the accelerated expansion of the Universe. This energy (density) makes around 69% of the cosmos and is called dark energy to account for the possibility that it might vary over time and space. For those of you more familiar with Physics, dark energy is not necessarily the constant introduced and thought to be related to the vacuum energy in quantum field theory (QFT). Dark energy remained a theory for around 14 years, until 1998 when the accelerated expansion of the Universe was indeed observed and in 2011 the Nobel Prize in Physics was awarded to Saul Perlmutter, Brian Schmidt, and Adam Riess “for the discovery of the accelerating expansion of the Universe through observations of distant supernovae”.
Additional observational evidence also comes from the Planck satellite launched in 2009 which obtained a power spectrum with an incredible accuracy for all cosmological parameters. Because of the Planck satellite, we now know with better than 1% accuracy that the Universe is 13.8 billion years old, the observational evidence for both dark energy and dark matter is stronger than ever. The measured anisotropies in the temperature of the CMB as measured by the Planck satellite contain all the key ingredients needed to better understand the Universe. By analyzing the angular size and the position of the first peak, cosmologists can conclude that the Universe is flat. By analyzing the relative height between the first and the second peak, cosmologists can conclude that ordinary matter makes only 5% of the Universe. By analyzing the third peak, cosmologists show that 26% of the Universe is dark matter and thus the remaining 69% (100%-5%-26%) must be dark energy.
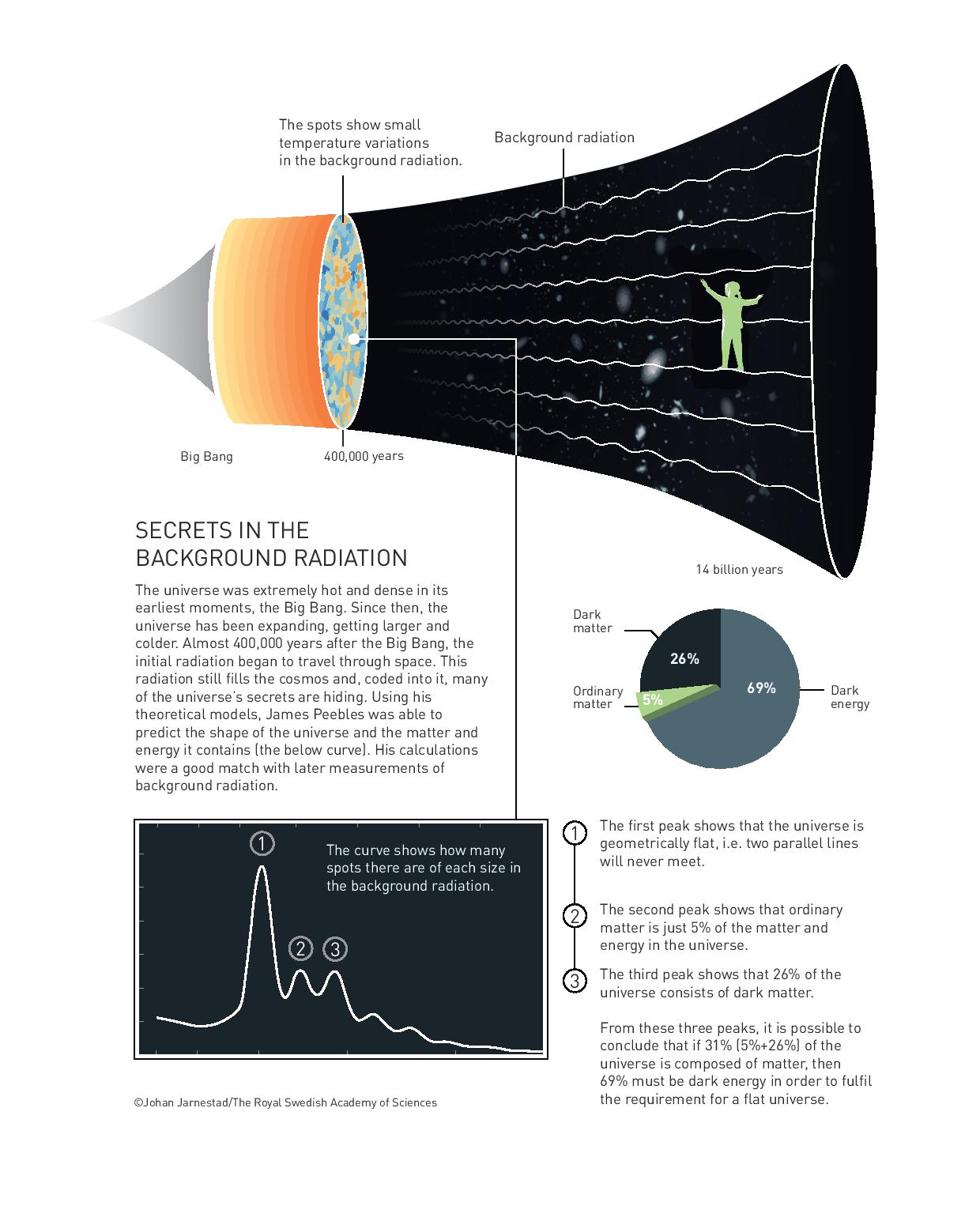
However, coming this far in cosmology, does not mean that we fully understand the physics behind dark energy and the nature of the dark matter. Perhaps the dark energy is varying with time, as suggested by Peebles, and we are still searching for suitable candidates for dark matter. It is indisputable that the theories postulated by Peebles fit the currently-available observational data with an astonishing precision, but there are also many other observations that await a full theoretical explanation. Nevertheless, these open questions in no way diminish the theories that Peebles developed over 20 years since these are precisely the theories that opened the doorway for cosmological theories and experiments to collaborate so effectively, that they eventually changed our view of the Universe.
I personally love it that there are unanswered questions, that we have something to look for, to question, to solve. The new era of Physics is here and it is more challenging and more thrilling than ever. It is not frustrating to keep on asking, to keep on searching; it is frustrating to think that we have found everything about the world we live in, and be fooled that we have understood the Universe and all of its greatness. A person is alive until she learns and oh, what a boring world it would be if we stopped learning.
Keywords:
Cosmic Microwave Background Radiation | Remnant electromagnetic radiation from the early stage of our Universe; the oldest electromagnetic radiation to our knowledge |
Black-body (radiation) | An object that absorbs all radiation falling on it, its emission is called black-body radiation. The concept is an idealization, perfect black bodies do not exist in nature. |
Cosmic Microwave Background Radiation Anisotropies | Small temperature fluctuations in the blackbody radiation left over from the Big Bang |
Open Universe | Universe with too little matter |
Dark Matter | 26% of the Universe, invisible matter whose gravity holds structures in the Universe – such as galaxies – together |
Cosmological Constant/Dark Energy | Energy of the vacuum, contributes to 69% of the Universe |

![Cai Jingxing: West Lake (1924) [1]](https://jungle-dancer.com/wp-content/uploads/2019/10/cai-jingxing_west-lake-75x75.jpg)
2 Comments
Pingback:
Pingback: